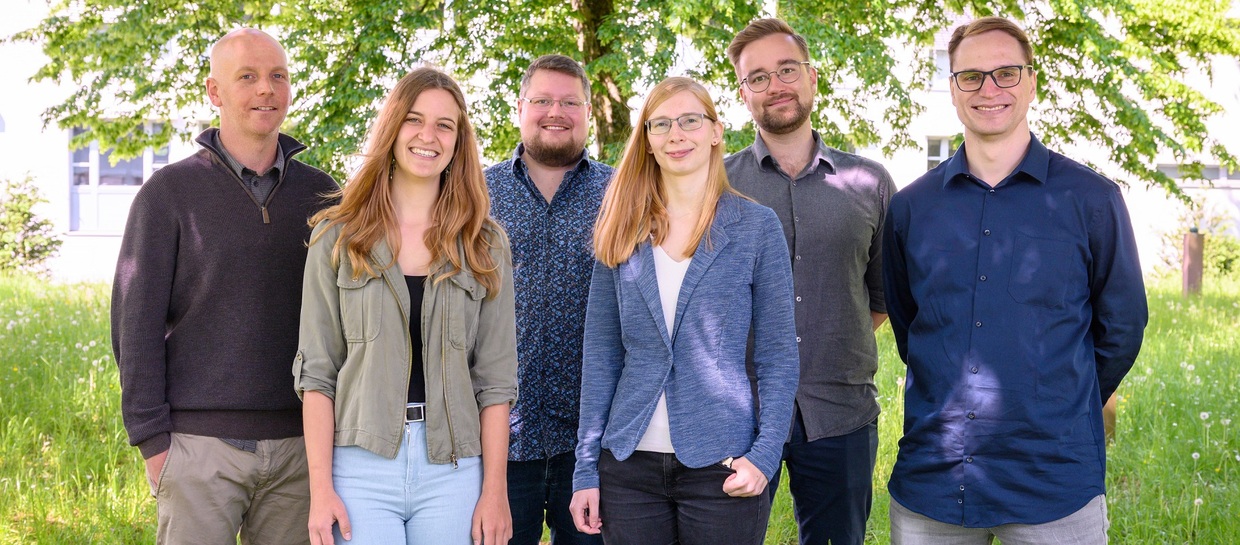
Being the energy carrier and transport medium, geothermal fluids are the key component for geothermal energy production. They are typically highly saline and have a complex composition, which is primarily governed by the reservoir rock type, temperature and pressure, residence time, and fluid origin. Dissolved gases, such as CO2, H2S, and CH4, can further add complexity. This difficult chemical environment poses challenges for the technical handling of the fluids at different stages along the thermal water circuit. Our research focuses on advancing in the fields of scaling assessment, raw material extraction, geochemical exploration, novel tracer development and flow path characterization.
Hydrochemistry of geothermal systems
Reliable quantification of the chemical system is essential for process understanding and predictions of the system’s behavior as a response to geothermal operations. Experimental techniques and numerical geochemical models are used to quantify the chemical interactions between rocks, fluids, gases and precipitates. The increasing availability of data enables us to use Machine Learning techniques to evaluate chemical systems based on encompassing chemical analyses. Novel approaches in this field are currently being developed in the MALEG project.
Heat extraction and depressurization during production change the physical, hydrochemical, and thermodynamic properties of geothermal water, disturbing its chemical equilibrium and may lead to scaling formation (mineral precipitation). We work on reliable scaling prediction, the design of processes for fluid treatment and scaling mitigation measures.
Raw materials dissolved in geothermal brines are increasingly becoming an interesting alternative to conventional deposits. Numerous constituents, e.g. lithium, boron, silica, and magnesium are contained in high concentrations. Making them more viable, a co-production of energy and raw minerals can create mutual benefits for both branches. As a low-invasive process, the extraction from brines opens up a more environmentally friendly alternative to conventional mining and can help to reduce Europe’s reliance on raw material supply from third countries. Our research focus in the BrineMine project is on the resource assessment, modeling of long-term reservoir behavior scenarios and the design of fluid treatment processes.
Depending on the play type and setting, suitable geochemical exploration methods are necessary to uncover key information about the reservoir, such as reservoir temperature and brine provenance for enabling sustainable reservoir management. We focus on creating novel techniques for determining reservoir temperature as well as advancing traditional geochemical exploration methods.
Recent and ongoing work
- Machine Learning for hydrochemical quantification in complex brine environments (MALEG project / Dissertation Michael Trumpp)
- Numerical models for scaling formation quantification (DeepStor project)
- Raw material extraction from geothermal brines (BrineMine project / Dissertation Valentin Goldberg)
- Development of exploration methods: New techniques for geothermometry (Dissertation Lars Ystroem)
- Geochemical exploration campaigns (e.g. Chile, Indonesia)
Flow paths in the reservoir
The performance of a reservoir is largely determined by its geometry, the spatial distribution of flow paths and their hydraulic properties. In geothermal reservoirs, often fluorescent dye tracers are injected and their recovery is measured to characterize the hydraulic parameters. In collaboration with the Institute of Nanotechnology at KIT, we are developing Smart Nanoparticle Tracers. We investigate transport and adsorption properties of these particle tracers and develop techniques to adjust them according to the specific requirements of the application. In addition to recovery over time measurements, more complex particle designs are currently studied which will allow also the detection of other reservoir parameters, e.g. temperature along the flow path.
Fluid flow in pre-existent and sheared fractures in a virtually dense rock matrix is characteristic of Enhanced Geothermal Systems. Geothermal-typical hydraulic boundary conditions together with the complex fracture geometries can cause turbulence-like flow behavior. In order to investigate the still unsolved central issues regarding hydraulics, transport and their impact on fluid chemistry in this context, we have established the DFG-funded F4aT hydraulic laboratory (Forced Fracture Fluid Flow and Transport). Utilizing a 3D scanner, a 3D printer and a hydraulic test set-up, turbulent flow regimes on rough fractures can be investigated experimentally. The results are used to improve process understanding and to calibrate and parameterize numerical models.
Recent and ongoing work
- Development and application of Smart Nanoparticle Tracers (Dissertation Laura Spitzmüller)
-
Experimental and numerical hydraulics in the F4aT – Laboratory (Dissertation Carola Buness)
Publication
Kombi: Name - Tätigkeit | Tel. | |
---|---|---|
Buness, Carola Marita Research associate |
+49 721 608-45290 | carola buness ∂does-not-exist.kit edu |
Yström, Lars Akademischer Mitarbeiter |
+49 721 608-45290 | lars ystroem ∂does-not-exist.kit edu |
Spitzmüller, Laura Research associate |
+49 721 608-41405 | laura spitzmueller ∂does-not-exist.kit edu |
Trumpp, Michael Research associate |
+49 721 608-45049 | michael trumpp ∂does-not-exist.kit edu |